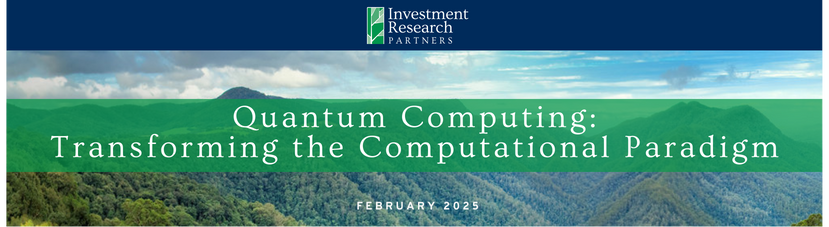
The emergence of quantum computing represents the potential for a profound leap in not just computing power but in the manner in which the world’s most powerful computers function to solve complex problems. Rooted in the principles of quantum mechanics, quantum computing redefines what is computationally possible, promising to solve problems that lie beyond the reach of even the most powerful classical computers. This paper explores the conceptual foundations of quantum computing, its distinctions from traditional computing, the leading organizations at the forefront of its development, and its far-reaching implications for industries and societies. We aim to provide a comprehensive understanding of this potentially transformative technology.
Think of a classical computer like a really fast and efficient light switch system. It processes information using tiny switches called transistors, which can be either on (1) or off (0). These on/off states are called bits, and everything your computer does—calculating numbers, displaying images, running programs—is built from billions of these switches flipping between 1s and 0s very quickly. A classical computer follows a step-by-step process to solve problems, like following a strict recipe.
Now, a quantum computer is more like a magic coin that can be both heads and tails at the same time—until you look at it. Instead of regular bits, it uses qubits (quantum bits), which can exist in multiple states at once due to a property called superposition. This means that instead of just being 0 or 1, a qubit can be some combination of both at the same time, allowing the computer to explore many possible solutions simultaneously rather than one by one.
In another analogy, classical bits can be likened to a thumbs-up or thumbs-down system, where a thumb pointing up represents a 1 and a thumb pointing down represents a 0. On the other hand, a qubit allows for the thumb to represent a value even if it’s not completely up or down. Thus, a thumb positioned at an angle which represents the qubit state, e.g., a 90-degree, or a 35-degree angle (in all directions), can also encode information. A thumb positioned horizontally represents a 0 and 1 at the same time. This paradigm allows the qubit to represent multiple states at once, leading to probabilistic measurement outcomes where the likelihood of measuring a 0 or 1 can vary based on the qubit’s state.
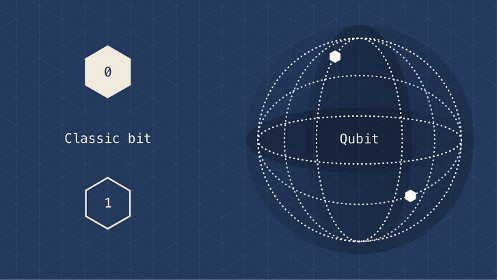
In quantum mechanics, superposition refers to a system existing in multiple states at the same time until it is measured. One common example is electron spin, where an electron’s spin can be either "up" or "down," but before measurement, it exists in a superposition of both states. This property is crucial in quantum computing, where spin states serve as qubits. Similarly, photon polarization can be horizontal or vertical, but until measured, a photon exists in both polarization states simultaneously, a principle used in quantum communication and cryptography.
Quantum computers have the potential to solve certain types of problems exponentially faster than classical computers. One of the most well-known applications is factoring large numbers, which is crucial for breaking encryptions. Classical computers struggle with integer factorization when dealing with very large numbers, but quantum computers, using Shor’s algorithm, can factor them exponentially faster, posing a significant threat to modern cryptographic systems.
Another area where quantum computing excels is in simulating quantum systems, particularly in materials science and drug discovery. Simulating molecules, proteins, and quantum interactions is infeasible for classical computers because the complexity grows exponentially. However, quantum computers naturally model quantum systems, allowing for much more accurate and efficient simulations, which could lead to breakthroughs in chemistry and medicine.
The Architecture of Quantum Computers
Quantum computers are fundamentally different from classical computers in both design and operation. A classical computer relies on bits, logic gates, and deterministic algorithms. In contrast, quantum computers use qubits, quantum gates, and probabilistic algorithms. Qubits can be implemented using various physical systems, including superconducting circuits, trapped ions, and photons.
Superconducting qubits, used by companies like IBM and Google, are created by passing currents through superconducting materials cooled to near absolute zero. These systems take advantage of the unique properties of superconductors to maintain quantum coherence. Coherence in quantum mechanics refers to the ability of a quantum system to maintain its quantum state over time without interference from the outside world. It’s what allows quantum particles, like qubits in a quantum computer, to stay in superposition and perform complex calculations. Remember, once the quantum element is observed or interacts with the outside world at all, it collapses into a single state and its superposition is eliminated. Environmental disturbances, such as thermal vibrations, electromagnetic interference, or even cosmic rays, can disrupt a qubit’s state, a phenomenon known as decoherence. Once a qubit loses coherence, the information it holds becomes irretrievable, leading to errors in the computation.
The Companies Leading Quantum Computing
The field of quantum computing is driven by both established technology giants and innovative startups. IBM has been a pioneer, with its IBM Quantum Experience providing cloud-based access to quantum processors. The company’s "Eagle" processor, unveiled in 2021, achieved a record 127 qubits, a significant milestone in the quest for scalability. IBM’s roadmap includes building systems with thousands of qubits, aimed at addressing real-world problems such as chemical simulations and optimization challenges.
Google’s quantum computing efforts achieved international attention in 2019 with its demonstration of quantum supremacy. The Sycamore processor solved a computational task in 200 seconds that would take the world’s fastest classical supercomputer thousands of years. Google continues to focus on scaling its systems and developing error correction technologies, aiming to make quantum computing a practical tool for scientific discovery and industrial applications.
Microsoft’s Azure Quantum platform integrates quantum computing into its broader cloud ecosystem. The company is exploring topological qubits, which promise greater stability and lower error rates. These qubits are based on theoretical constructs that minimize decoherence, potentially revolutionizing the scalability of quantum systems. Similarly, Rigetti Computing focuses on hybrid quantum-classical systems, offering solutions that combine the strengths of both paradigms. Their cloud-based quantum platform enables businesses to experiment with quantum algorithms alongside traditional computing resources.
Startups like IonQ and PsiQuantum are also making significant strides. IonQ’s trapped-ion approach has demonstrated high precision and scalability, while PsiQuantum is pursuing a photonic quantum computer designed to operate fault-tolerantly. PsiQuantum’s vision involves leveraging photonic systems to create a million-qubit system capable of solving complex problems in areas like drug discovery and materials science. Chinese technology firms such as Alibaba and Baidu have also invested heavily in quantum research, reflecting national priorities in emerging technologies. The race for quantum leadership is shaping up to be a global competition with profound implications for technological and economic dominance.
Applications Across Industries
The potential applications of quantum computing span numerous industries, each benefiting from its unique computational capabilities. In healthcare, quantum simulations enable precise modeling of molecular interactions, accelerating drug discovery and personalized medicine. For example, simulating protein folding, a task that stymies classical supercomputers, could be revolutionized by quantum algorithms.
In finance, quantum algorithms optimize portfolios, enhance risk modeling, and detect fraudulent transactions with greater efficiency. Financial institutions are exploring how quantum computing can improve derivatives pricing, arbitrage strategies, and credit risk assessments. The ability to process vast amounts of financial data simultaneously offers a competitive edge in an industry driven by speed and precision.
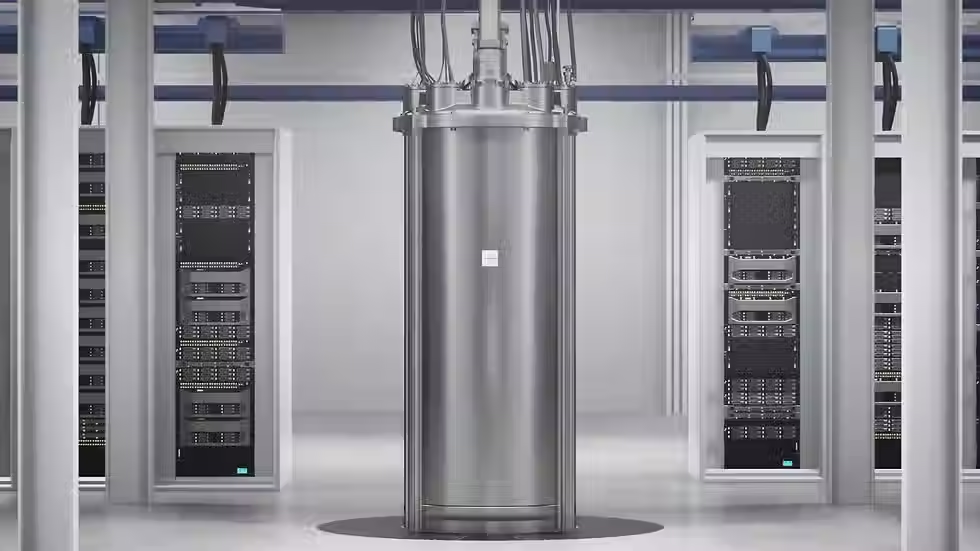
The energy sector stands to benefit from quantum computing through the optimization of power grids and the development of new materials for batteries and solar panels. Quantum algorithms can analyze and optimize complex networks, reducing energy waste and improving sustainability. Climate science can leverage quantum models to better understand complex environmental systems, aiding in the prediction and mitigation of climate change. By simulating atmospheric conditions and ocean currents with unprecedented accuracy, quantum computing could play a critical role in addressing global warming.
Even logistics and supply chains, industries reliant on optimization, can achieve significant efficiency gains through quantum solutions. Companies like DHL and Volkswagen are exploring how quantum algorithms can optimize delivery routes and reduce transportation costs. Quantum-enhanced scheduling and resource allocation promise to transform operations in industries ranging from manufacturing to air travel.
The Challenges Facing Quantum Computing
Despite its promise, quantum computing faces formidable technical and practical challenges. Chief among these is the issue of error rates. Quantum systems are highly sensitive to their environments, and even slight disturbances can cause decoherence, disrupting computations. Developing error-correction techniques and stabilizing qubits are critical hurdles.
Scalability is another challenge. While current systems operate with tens or hundreds of qubits, practical quantum computing will require millions of error-corrected qubits. Achieving this scale demands advances in materials science, engineering, and algorithm design. Researchers are exploring new materials and architectures to improve qubit coherence and reduce error rates. Additionally, the high costs of building and maintaining quantum systems, which often require cryogenic conditions, limit their accessibility.
Conclusion
Quantum computing represents a paradigm shift in our approach to computation. By harnessing the principles of quantum mechanics, it transcends the limitations of classical systems, unlocking new possibilities across industries. While significant challenges remain, the progress achieved by leading companies and research institutions underscores the transformative potential of this technology. As quantum computing advances, it will reshape industries, economies, and societies, making it imperative for investors to understand and engage with its development.
References
Nielsen, M. A., & Chuang, I. L. Quantum Computation and Quantum Information. Cambridge University Press, 2010.
Preskill, J. "Quantum Computing in the NISQ Era and Beyond." Quantum, 2018.
Arute, F., et al. "Quantum Supremacy Using a Programmable Superconducting Processor." Nature, 2019.
IBM Quantum. "What is Quantum Computing?" Accessed January 2025.
Rigetti Computing. "Hybrid Quantum-Classical Computing Solutions." Company Website, 2025.
Important Disclosures
Past performance may not be representative of future results. All investments are subject to loss. Forecasts regarding the market or economy are subject to a wide range of possible outcomes. The views presented in this market update may prove to be inaccurate for a variety of factors. These views are as of the date listed above and are subject to change based on changes in fundamental economic or market-related data. The ETFs presented above are not intended to be benchmarks for performance. Rather, they are intended to be demonstrative of a particular sector or segment the investment universe discussed. Each ETF was selected as opposed to an index to more accurately reflect what an investor might experience. There are other ETFs or indices that might be representative of the same spaces. However, we believe the ones shown are sufficiently representative to assist us in explaining our investment thesis. Please contact your Advisor in order to complete an updated risk assessment to ensure that your investment allocation is appropriate.

Commenti